ACE OF GOLD
NEURONS
Introducing the Ace of Gold of the neurodeck: neurons. We have chosen neurons to represent the suit of gold in our neurodeck because they are million-dollar cells, rich and very valuable.
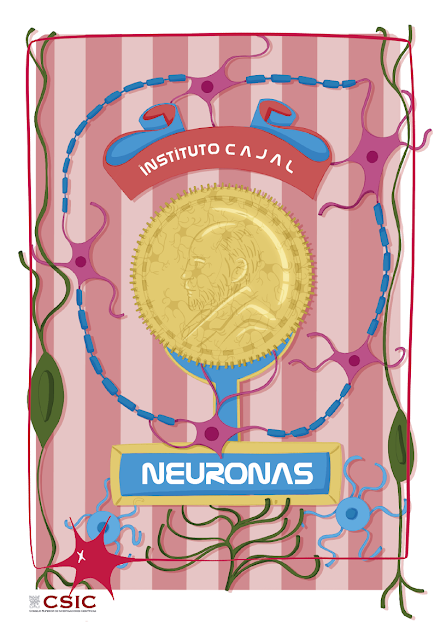
Neurons are millionaires due to their number: the latest estimates based on the Suzana Herculano-Houzel method put the number of neurons in an adult human brain at 86 billion. They are also millionaires for the number of connections they can establish: it seems that each neuron can receive information through about 10,000 synapses and send it through another 1,000. They are rich in forms, structures and functions. And they are very valuable, because they control our vital functions and our response to environmental conditions. Join us to discover them!
In the Animal Kingdom, the nervous tissue assumes the relationship function of the organism, which allows it to collect information from the external and internal environment to process it, integrate it and respond accordingly. Not all animals have neurons (sponges, mesozoans, and placozoans lack them) and not all nervous systems are equally complex. The most developed is that of vertebrates, but in its simplest form they can be simple networks of neurons distributed throughout the animal’s body.
In vertebrates, the neurons and glia cells of nervous tissue group together to form the central nervous system—composed of the brain structures housed in the skull (cerebrum, cerebellum, and medulla oblongata) and the spinal cord that runs through the interior of the spinal column—and the peripheral nervous system—made up of a network of nerves and ganglia that carry the nervous impulse throughout the body. Both systems are coordinated, allowing animals to capture stimuli through their sensory organs and emit signals to communicate with the outside world, maintain the balance of their internal environment (homeostasis) and send information to the muscles to move them. And all this is possible thanks to neurons, which are the functional information processing units.
Knowledge of the nervous tissue, and neurons in particular, took a spectacular leap as a result of the research of Santiago Ramón y Cajal, that versatile Spanish doctor who, at the end of the 19th century, intuited that nervous tissue stained with the “reaction black» [1] Golgi did not form a continuous nervous network that functioned as a whole, as many scientists thought at the time. Cajal deduced from his microscopic observations that neuronal networks were made up of independent cells that “kissed” each other to transmit information in the form of a nervous impulse.
With his optical microscope, Cajal was able to conceive the neuron doctrine [2], which revolutionized the scientific panorama of the time, laid the foundations of modern neuroscience, and justified his receiving the Nobel Prize in Physiology and Medicine in 1906 ( curiously shared with Camillo Golgi, defender of the opposite reticularist theory). Half a century later, technological advances proved Cajal right after observing the ultrastructure of nervous tissue with the electron microscope and confirming that neurons were physically separated by a space up to 3,500 times thinner than a hair (20 to 40 nanometers). , called the synaptic space.
It was Wilhelm von Waldeyer-Hartz who called this nerve cell a “neuron” in 1891, in clear support of the neuronal theory. Six years later, Charles Scott Sherrington (our neurodeck king of clubs😉) would call the points of contact and transmission of information between adjacent neurons synapses, and would receive the Nobel Prize in Physiology or Medicine in 1932 for his findings on the functions of neurons.
In any neuron, three parts are distinguished: a thin axis or “axon” that emerges from the “soma” or cell body (with the nucleus and cytoplasmic organelles) surrounded by branches or “dendrites.” However, neurons are very diverse (they can have a soma of 5 micrometers or giant axons with a diameter of one millimeter) because they perform complicated communication and regulation functions that require categorizing and associating information to process and transmit it as quickly and efficiently as possible. in the form of electrical and chemical signals. To adapt to the place where they are located and the function they perform, the size and shape of the soma, the density, the characteristics (with more or fewer spines) or the organization of the dendrites, as well as the arrangement, length or the type of axon branching are different for each type of neuron.
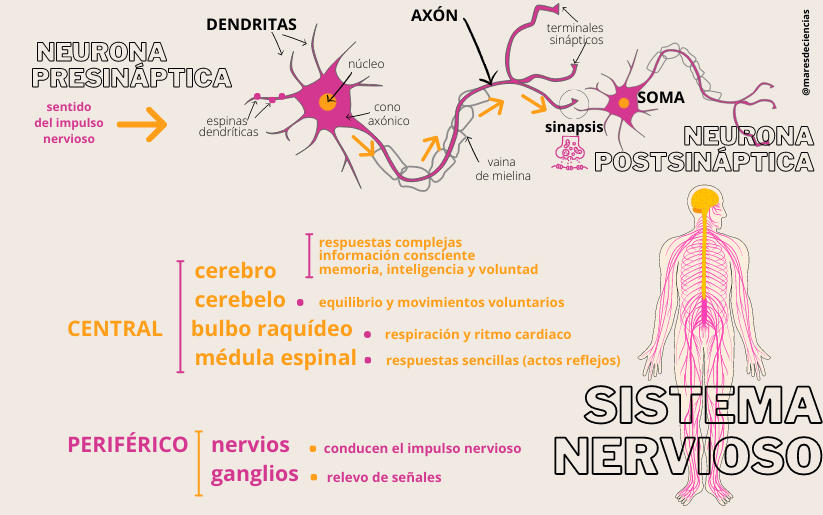
The classification of the different types of neurons can be done based on different criteria:
Because of the effect of one neuron on another, neurons can be excitatory, inhibitory, or neuromodulatory (when they influence neurotransmission).
By the type of neurotransmitter they release to communicate with each other (e.g., dopaminergic neurons release dopamine, cholinergic neurons release acetylcholine, etc.).
Due to the number of processes on the soma, neurons can be unipolar, pseudounipolar, bipolar, multipolar or anaxonic.
Due to the shape of the cell body, there are pyramidal, stellate, candelabra, basket, fusiform, spherical neurons…
Due to the presence of spines on their dendrites, neurons can be spiny or not.
Depending on the target cell they contact, neurons are sensory when they communicate the sense organs (e.g., skin or eye) with the brain or spinal cord, motoneurons if they communicate the brain and spinal cord with receptor organs (e.g., muscles or glands), or interneurons that connect neurons in the brain (unrelated to sensory or receptor organs). Sometimes communication with muscle cells is through specialized synapses called motor end plates.
Depending on the length of their processes, projection neurons establish synapses with very distant neurons and interneurons contact nearby neurons.
The functioning of the neuron is based on the electrical and chemical mechanisms of its plasma membrane [3] that make it excitable and conductive. Alan Lloyd Hodgkin and Andrew Huxley proposed a mathematical model in 1952 to explain the ionic mechanisms that initiate and propagate the nerve impulse, thanks to their work with the giant squid axon (which can have a diameter of up to a millimeter). In 1963, both shared the Nobel Prize in Physiology or Medicine with Sir John Eccles, who worked on the chemical transmission of nerve impulses in synapses.
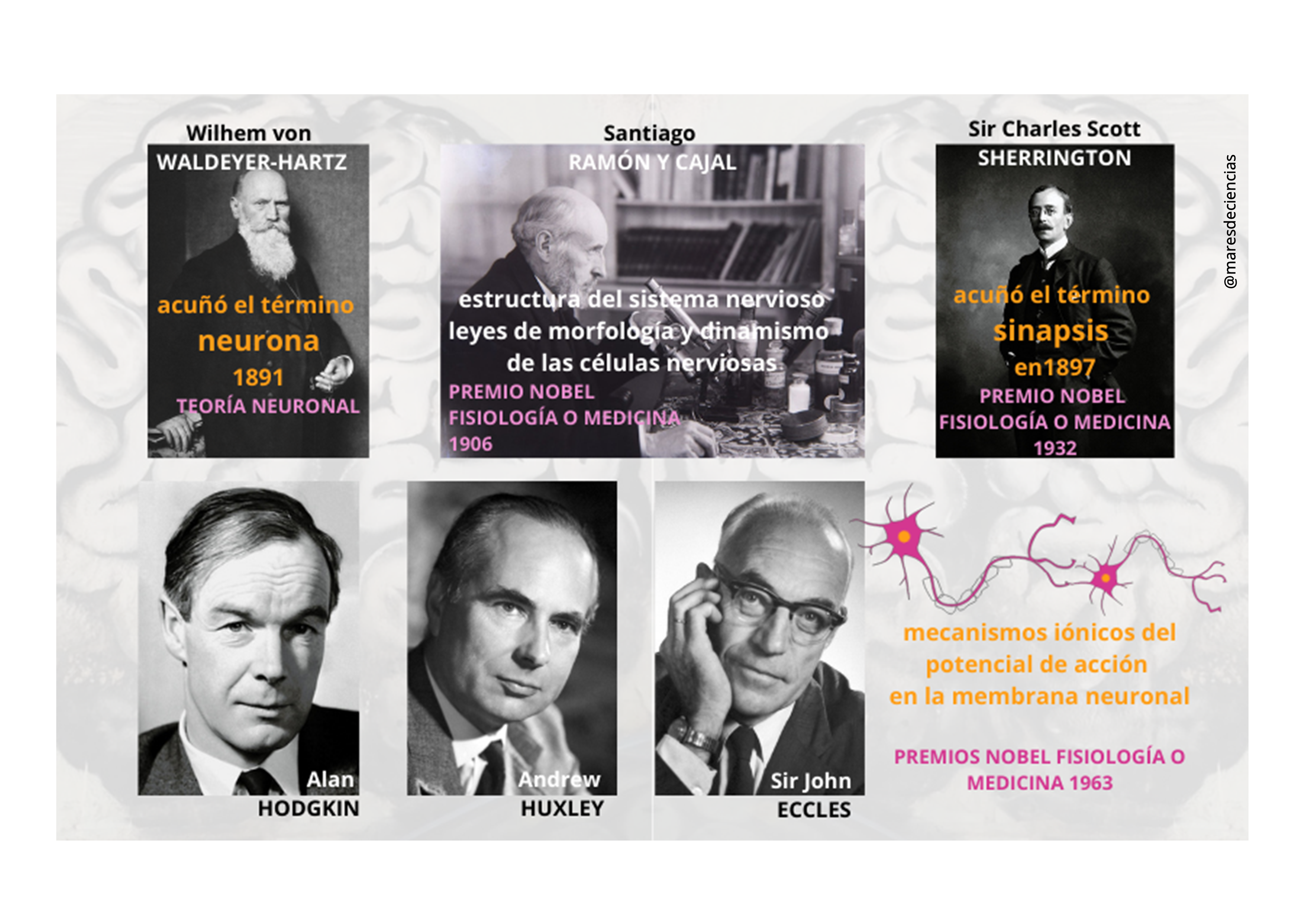
Thus, nerve impulses are intense but transient changes in membrane potential that move along the axon and always flow in a single direction, as Cajal discovered: from the axon cone, where they are generated, to the synaptic terminals, where produce their effects (it is the “law of dynamic polarization of nerve cells” [2]). Action potentials are signals that are generated and sent, at a high frequency, in series or “trains” along axons [5]. After launching an action potential, the neuron is no longer receptive to new depolarizations and cannot transmit them for a few tenths of a second (refractory period) until it returns to its resting potential.
Depolarization occurs when the resting neuron receives a chemical, temperature, pressure, or electrical stimulus that triggers the massive influx of positive ions into the neuron. However, the stimulus must be strong enough to reach a minimum threshold (set at —55 mV) that initiates an action potential. That is why it is said that the transmission of the nervous impulse follows the “law of all or nothing.” Furthermore, since the nervous impulse that is produced always has the same intensity (it does not increase or decrease), the greater or lesser strength of the stimulus can be achieved. increasing the frequency or speed of transmission.
To speed up the conduction of the nerve impulse, some neurons have their axons partially covered with myelin, an insulating substance that favors the “jumpy” transmission of the nerve impulse. In the brain and spinal cord, this myelin coating is produced by glial cells called oligodendrocytes (which we will talk about in future posts), while in the ganglia and nerves, Schwann cells are responsible for doing so.
We now know how neurons translate stimuli into nerve impulses thanks to the electrophysiological phenomena described by Hodgkin and Huxley, but this would be useless in the nervous system without another fundamental characteristic of the neuron: its ability to interconnect with similar cells thanks to specializations. in their cell membranes called synapses. However, in addition to the synapses between neurons, the latest research speaks of also considering the active connections between neurons and glial cells, as well as between glial cells and neurons [6].
This ability to create neural circuits is what allows them to analyze and preserve complex information, to carry out sophisticated functions associated with intelligence, memory and will. The specialization of the nervous tissue at the service of its transmission needs is such that neurons are capable (with the help of glial cells) of reorganizing their connections by remodeling the dendritic and axonal trees (synaptic pruning), or of varying the number or properties of the synapses that connect them.
The structure where two adjacent neurons exchange information is made up of three elements (tripartite synapse, a concept discovered by researchers at the Cajal Institute): the presynaptic or sending neuron, the postsynaptic or receiving neuron and an astrocyte, which is the glial cell that surrounds the set and is known as the “third element.”
In the human body, the vast majority of synapses are “chemical synapses.” They are called that because, when, after traveling through the branches of the axon, the depolarization wave reaches the axon terminals, it causes the release of neurotransmitters into the synaptic space.
Neurotransmitters are substances produced by neurons to carry messages of excitation or inhibition to provoke, or not, a new electrical impulse in the postsynaptic neuron. Currently there are about 50 different neurotransmitters known. The existence of chemical synapses means that information travels through controlled, very precise and selective circuits, although it does so more slowly than in electrical synapses.
Electrical synapses allow faster communications:
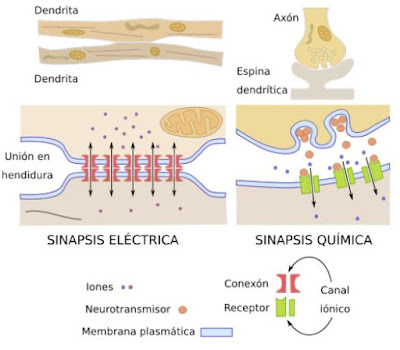
Scheme showing the differences between an electrical and a chemical synapse. Source: MEGÍAS, Manuel, Pilar MOLIST and Manuel A. POMBAL. Atlas of Plant and Animal Histology. Cell types. Neuron (February 2018 version). Department of Functional Biology and Health Sciences. Faculty of Biology of the University of Vigo
As you see, neurons are a treasure. But be careful! because this treasure is completed by the glial cells, those numerous but ignored companions of the neurons, in whose framework they are integrated and without whose support the neurons could not function correctly. We will talk about them in the next publications. Stay tuned!
NOTES
[1] The “black reaction” (reazione nera, in Italian) is a method for dyeing fabrics developed by Camillo Golgi, who shared the Nobel Prize with Cajal in 1906, and which the Spaniard used to achieve in 1874 something impossible until then. : observe the entire structure of the cells of the nervous system including the cell body, the axon and the branching dendrites of the neurons. (Neuroscience, José Ramón Alonso’s blog, entry “Golgi and the black reaction” 03/26/2014: https://jralonso.es/2014/03/26/golgi-y-la-reaccion-negra/
[2] The neural theory, which Cajal summarized in several articles, of which the most notable is Neuronism or reticularism? (1933), is based on four basic principles: the neuron is the main structural and functional unit of the brain; the axon terminals of one neuron communicate with the dendrites of another at specific locations (later named “synapses” by Charles Sherrington); Neurons connect to each other at specific sites to configure circuits, and nerve signals follow a predictable flow in the same direction: they enter through the dendrites towards the cell body and leave through the axon (“Law of dynamic polarization of cells “nervous”). Cajal also dictated the laws regarding the morphology and dynamism of nerve cells that explain the utilitarian significance of their various forms and associations, which he included under the title of “Laws of saving space, time and conductive matter.” of discovering and enunciating the “Principle of Divergence”—principle of “Avalanche of Conduction” he called it—by which a single nerve cell can activate or inhibit a population of neurons that come into contact with the first.
https://cvc.cervantes.es/ciencia/cajal/cajal_recollections/introduccion_15.htm
[3] The “semipermeability” of the plasma membrane of any cell allows the composition inside (intracellular) and outside the cell (extracellular) to be different thanks to the fact that it only allows certain molecules or charged atoms (ions) to pass through. In the cell membrane there are proteins that cross the membranes (transmembrane proteins) or ion channels that allow ions to enter or leave the cell along the electrochemical gradient (without requiring energy). When the transport of ions to one side or the other of the cell must be done selectively against a gradient, energy and the intervention of ion pumps are required (such as the Na/K pump, which removes three sodium ions and introduces a potassium ion to maintain the resting membrane potential).
[4] The outside of the neuronal membrane has positive electrical charges, while the inside is dominated by negative electrical charges. This difference is due mainly to the higher concentration of sodium (Na+) and chlorine (Cl—) ions in the tissue fluid that bathes the nerve cell, and to the many potassium ions (K+) and large “negative organic ions.” », in the cytoplasm of the neuron.
[5] PÉREZ IGLESIAS, Juan Ignacio. Giant neurons. Published on 11/19/2015 on the Zoo Logik blog http://zoologik.naukas.com/2015/11/19/neuronas-gigantes/
[6] A 2009 work showed that neurons not only establish synapses among themselves, but could do so with glia cells, such as those identified as NG2 (BERGLES DE, JABS R, STEINHÄUSER C. Neuron-glia synapses in the brain. Brain Res Rev. 2010 May; 63(1-2):130-7. doi: 10.1016/j.brainresrev.2009.12.003). Also a work from 2014 establishes the existence of communication between neurons and other glial cells, such as astrocytes (MARTÍNEZ–GÓMEZ, A. Communication between glial cells and neurons I […] Journal of Medicine and Research 2014; 2(2 ): 75-84 https://www.elsevier.es/es-revista-revista-medicina-e-investigacion-353-pdf-S2214310615300029). Research on neuroglial cells is a very active field that is gradually increasing our knowledge about the types of cells that make it up and their functions, although many unknowns still remain.
SOURCES
DE FELIPE, Javier. Cajal and neural circuits (Cajal Institute – CSIC)
http://www.banquete.org/banquete08/Cajal-y-los-circuitos-neuronales
GODWIN, Dwayne, Jorge CHAM and Meg ROSENBURG. Mind a minute: your brain by the numbers https://www.youtube.com/watch?v=0DuPzbYsCig
MEGÍAS, Manuel, Pilar MOLIST and Manuel A. POMBAL. Atlas of Plant and Animal Histology. Cell types. Neuron (February 2018 version). Department of Functional Biology and Health Sciences. Faculty of Biology of the University of Vigo.
Animal tissues. Nervous tissue https://mmegias.webs.uvigo.es/guiada_a_nervioso.php
Cell types. The neuron https://mmegias.webs.uvigo.es/descargas/tipos-cel-neurona.pdf
About the number of neurons in the human brain:
ZORZETTO, Ricardo (2012) Revised numbers. Counting neurons puts neuroscience in check. Pesquisa FAPESP Magazine edition 192, February 2012 https://revistapesquisa.fapesp.br/wp-content/uploads/2012/02/026-031_Neuro_espanhol_2.pdf
HERCULANO-HOUZEL, Suzana. What is so special about the human brain? TEDGlobal talk 2013 (video with transcript available in Spanish: What is so special about the human brain? ) https://www.ted.com/talks/suzana_herculano_houzel_what_is_so_special_about_the_human_brain/transcript
VON BARTHELD, Christopher S., Jami BAHNEY, Suzana HERCULANO-HOUZEL (2016). The search for true numbers of neurons and glial cells in the human brain: A review of 150 years of cell counting. The Journal of Comparative Neurology, vol.524 (18), pp. 3865-3895. https://doi.org/10.1002/cne.24040
At the Cajal Institute, the Cellular Electrophysiology Group studies “the cellular mechanisms [such as the electrical properties of the membranes of excitable cells] that intervene in the processing of information that underlies the processes of memory and learning”
BUÑO, W. and A. ARAQUE (2006): Characteristics of the cellular components of nervous tissue: electrical properties of the membranes of excitable cells. Section II, topic 4 of the Master’s Degree in Neuroscience and Behavioral Biology 2007 (National Administration of Public Education of Uruguay). Viguera Editores, S.L. pp. 147-173. http://www.anep.edu.uy/ipa-fisica/document/material/primero/2008/espacio/propelec.pdf
Membrane theory: http://www.facmed.unam.mx/historia/TeoriaMembrana.html
Neuroscience for dummies. YouTube Channel of the Cajal Institute in collaboration with AsociaciónConCiencia
The brain dictionary. YouTube Channel Cerebrotes, by Clara García
Neurons https://www.youtube.com/watch?v=mT3wJGB_BEY&ab_channel=Cerebrotes
Axon https://www.youtube.com/watch?v=QMP9a_zih8s
Soma https://www.youtube.com/watch?v=0kCUgF0WOd0
Dendrites https://www.youtube.com/watch?v=8YGa8bPBz80
What is the action potential https://www.youtube.com/watch?v=TAnhxsP-HlY
What are synapses https://www.youtube.com/watch?v=DEzT_JM6WfY&t=2s
What are neurotransmitters https://www.youtube.com/watch?v=qkYCGALqS-E&t=4s
Neurostories. YouTube Channel Cerebrotes, by Clara García.
When was the first neurotransmitter discovered? https://www.youtube.com/watch?v=z2Bn1yfXcmA&t=8s
UnProfesor YouTube Channel
What is synaptic integration https://www.youtube.com/watch?v=FZ6YrPo0qxM
Chemical synapses and electrical synapses https://www.youtube.com/watch?v=6nGxTPo_lvg
Neurotransmitter release https://www.youtube.com/watch?v=3S91NVZ0rkk
The refractory period https://www.youtube.com/watch?time_continue=10&v=jAt5MEwoDkQ&feature=emb_logo
Classification of neuron types https://lasneuronascolfem.blogspot.com/p/clasificacion-de-neuronas.html
INTERACTIVE TEST TO REVIEW WHAT YOU KNOW ABOUT NEURONS